Table of Contents (click to expand)
The Wave-Particle Duality states that an electron can be described as both a particle and a wave. This means that an electron can exhibit the properties of both a particle and a wave depending on the situation. For example, an electron can behave like a particle when it is fired from the nozzle of a LASER, but it can also behave like a wave when it is passed through a double slit.
Light was thought to be a wave wafting through spacetime, like a ripple in a pond, until 1905, when Einstein showed that it also behaves like a particle. This was a startling revelation. Einstein had finally solved a curious physics problem of how light incident on a metal would knock electrons out from its surface. He realized that this would only be possible if light did not behave like a wave, but rather like rigid stones, or discrete packets of photons, as he called them. The light particle would knock out electrons in the same way that a cue ball knocks an 8-ball. Despite depicting something as mind-boggling as time-travel, Einstein won the Nobel Prize for this discovery – for explaining the photoelectric effect.
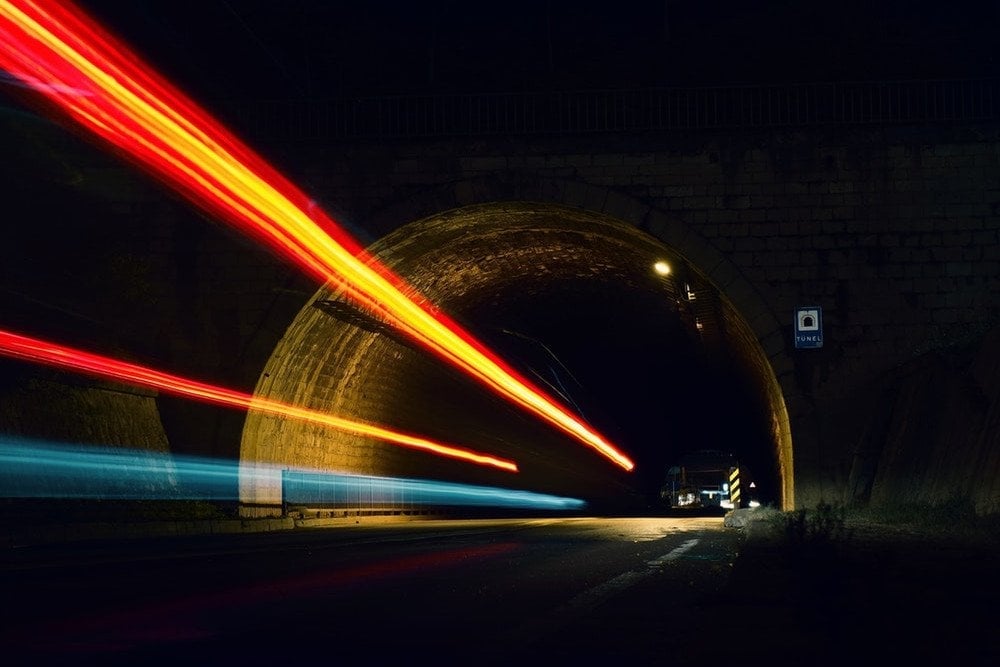
With this discovery, Einstein sparked what is now called the Wave-Particle Duality. Light leads a dual life — it exists as a wave when the luminance of a candle flows around an object that obstructs its path, but it also exists as a particle when fired from the nozzle of a LASER. However, is it just light that is endowed with this fickle personality? Why would nature encourage such asymmetry? Or, does matter also exist in ripples? These were the questions raised by Louis de Broglie in his Ph.D. thesis in 1924. What did he conclude? Yes, matter also behaves like waves.
Recommended Video for you:
The Double Slit Experiment
De Broglie’s thesis revolutionized modern physics. Matter’s duality inspired the creation of an entirely new branch of modern atomic physics called wave mechanics, an esoteric field that forms the very roots of quantum mechanics. Does this mean that we can also flow around objects like the silver, slimy antagonist with the physiognomy of an academy award in Terminator 2? Technically, yes. Consider the ingenious experiment that proved de Broglie’s hypothesis.
Davisson and Garner performed a landmark experiment showing that when electrons are fired towards two slits, the pattern generated on a fluorescent screen placed in front of them replicates an interference pattern. An interference pattern is to waves what coldness is to ice. An interference pattern is basically the identity of waves. When monochromatic light (light of a single wavelength) passes through two slits, some waves combine, while the others cancel each other out to form a long alternate pattern of light and dark bands on a screen ahead.
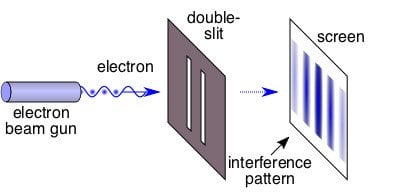
However, if you were to send multiple tennis balls through the two slits, each tennis ball would simply go through either of them, thereby forming on the screen only two bands of tennis balls. This is what you’d intuitively expect from particles, such as, well, electrons. However, this is not the case. Davisson and Garner showed that when you send electrons through the two slits, they illuminate the screen with not just two, but a long alternate pattern of light and dark bands of electrons!
Also Read: What Is The Double-Slit Experiment?
Louis De Broglie’s Hypothesis
Imagine the shock of the experimenters. This is highly counter-intuitive, but it’s true. Subsequent experiments have consistently proved de Broglie’s preposterous claim. Matter, oddly, really does possess a wavelength. De Broglie found this wavelength ‘λ’ to be the ratio of h/p, where ‘h’ is Planck’s constant, which has a value of , while ‘p’ is the momentum of the object.
He arrived at this relation by equating the energies of the two asymmetric systems that he believed were actually symmetric: light’s energy pc and the discrete energy of a single photon hf. Now, c = f λ, which gives the relation: p λ = h or λ = h/p.
Now, because the hypothesis is true for an electron, it is also true for anything that electrons compose – which is basically everything. Let’s consider an orange basketball. The reason a basketball does not waver and flow around objects is that the wavelength associated with it is infinitesimal. Plug in the values and see it for yourself. No matter how massive it is or how fast it moves, it cannot outweigh the quantity in the numerator. The wavelength of one basketball measures in the scale of meters.
The calculation of the de Broglie wavelength associated with a 1kg basketball.
The double slit experiment can only be conducted if the wave’s wavelength is comparable to the width of the slits. If you were to replicate Davisson and Garner’s result on a basketball, you’d require slits that were a width of meters. The current technology does not permit this. However, an electron’s wavelength measures on the scale of
meters. This is due to an electron’s paltry mass and swift velocity. The development of slits this wide is permitted by current technology.
Essentially, a basketball does possess a wavelength, but it far too small to be perceived. Not just a basketball, but also you, me and everything in the entire Universe exhibits a wave-particle duality. Nature is, after all, symmetric.
Also Read: Without Magic, Could You Get Through Platform 9 ¾?
The Mystery That Is Quantum Mechanics
At first, I, like everyone else, was baffled. All these years of schooling had cemented my particle interpretation of an electron. How is it possible that the rigid electrons flow around the slits and draw an interference pattern? Do they split before they enter and coalesce after they exit? Does the Universe even make sense?
Skeptics speculated that the pattern was a result of the clamor of electrons barging through the slits. To test this, experimenters conducted the experiment by transmitting the electrons through the slits one at a time, like a trail of obedient ants. However, to their ultimate astonishment, the pattern still emerged!
Their finding blatantly belies our perception of rigidity. Fine, I will let go of my vestigial views, but how must I imagine ripples of electrons? This contradiction, this inability to comprehend an electron wave in the traditional sense of waves, troubles us deeply. Fortunately, one can find respite (or delirium) in the fact that these waves aren’t the traditional ripples we find in a pond, but rather ‘probabilistic’ waves. While one can locate the stone that causes a ripple, the same cannot be said about an electron.
For a probabilistic wave, the stone cannot be located, as its location is uncertain. One cannot explicitly say it is ‘here’ or ‘there’. What one can say is that the stone is more ‘probable’ to be found here or there. In a way then, it is ‘everywhere’, its location is ‘distributed’. However – and this is why I appended respite with delirium – this notion of probabilistic waves implies that the electron passes through both slits at the same time!
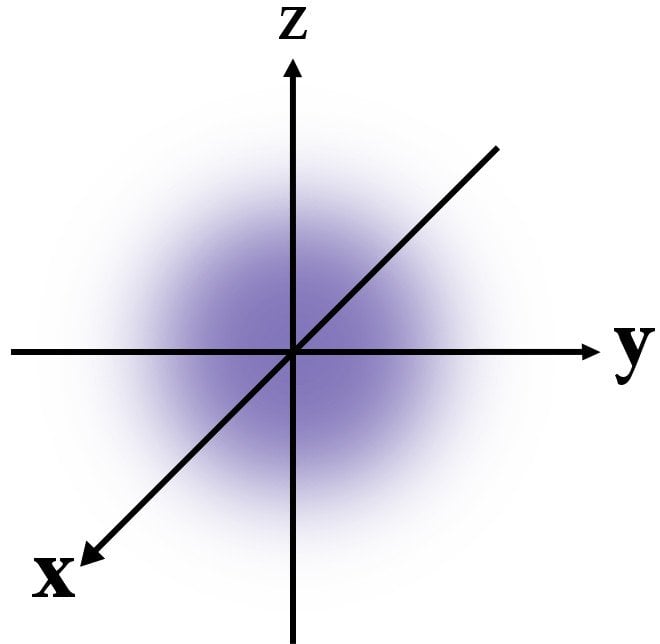
The physicists were relentless. They wanted to see this ‘distribution’ with their own eyes. They planted scrupulous detectors that would surveil an electron as it passed through the slit. What they observed stupefied them. Now that they observed each and every passing electron, the image on the screen assumed the predicted pattern of a particle or tennis ball! The resulting pattern simply comprised two vertical bands replete with electrons. At this point, everyone put up their hands in helplessness and likely exclaimed, “We’ve had enough.”
What happened was that the mere act of observation altered the nature of the electrons irreversibly. Two concepts explain this absurd behavior, two concepts that non-scientific culture so readily identifies with the mysteries of the quantum world: Heisenberg’s uncertainty principle and Schrodinger’s unfortunate cat.
Analyzing the dual nature of electrons is like listening to a song: when you are looking forward to appreciating the lyrics (location), you lose track of the beautiful piano, the soft guitar and periodic bongos, the essential tunes (momentum) that constitute its melody. Whereas, when you are determined to appreciate the tunes, you lose track of the heartfelt lyrics. We cannot lend our devices to a single aspect; we must attain a suitable compromise to appreciate the music as a whole.
In the experiments without those delicate detectors, we are highly uncertain about the electron’s exact location and highly certain about its energy and therefore, momentum. In the realm of quantum mechanics, momentum is equated with distribution or wavelengths or waves, while location is equated with exactitude or particles. The knowledge of momentum therefore causes an electron to behave like a wave. Whereas, in the experiments with the detectors, we are highly certain about the electron’s location and uncertain about its momentum. This act of observation forces it to behave like a particle. The changes in its nature are, as mentioned, irreversible. The cat is both dead and alive until you open the box to see it for yourself. Spooky.
In short, in conjunction, the electron is both a particle and wave until we measure either of its defining characteristics — momentum or location. Once either of them is measured, its nature is permanently decided.
De Broglie won the Nobel Prize for his profound discovery, whose implications later became central to the plots of science fiction. In the real world, de Broglie’s finding is a grave threat to Moore’s law, which states that transistors implanted on a semiconductor substrate must double every year. Currently, in the range of nanometers, the wires would eventually become comparable to the size of electrons, allowing intrinsic quantum effects to mess with their operation. Engineers wonder how small the wires must be before the electrons flow around the apparatus like they do the around slits.
However, the wave nature of electrons also paved the way for the development of electron microscopes. These microscopes don’t illuminate samples with light, but rather electrons. The waves of electrons are then magnified by powerful magnets, just as the waves of light are magnified by lenses. They can achieve a magnification of up to 10,000,000 times. This has allowed microbiologists and chemists to study molecules in stunning detail. Again, our traditional interpretation of waves is muddled, yet as Neil deGrasse Tyson claims: “The Universe is under no obligation to make sense to you.”
Also Read: Why Is Quantum Mechanics So Difficult To Understand?