Table of Contents (click to expand)
The pioneering research and development in the nuclear domain was performed with the purpose of creating nuclear weapons. Since the fission of thorium doesn’t produce plutonium (one of the elements used in nuclear weapons) as a byproduct, uranium, the dual-purpose nuclear fuel, took precedence. However, nations have finally begun to understand the safety aspects of thorium as a nuclear fuel and have started developing thorium-based nuclear reactors.
After recently binging on the show Chernobyl, I found myself deep in the rabbit holes of Google, digging into the mysteries of nuclear reactors and how they work. I soon discovered that modern human history is filled with nuclear accidents. Since the inception of the very first nuclear plant in 1954, there have been more than 100 serious nuclear accidents, each affecting the lives of thousands—or even millions!—of humans and animals.
At the crux of these accidents is uranium, the element used as the primary nuclear fuel in all nuclear reactors built to this point. To my surprise, however, I found that there is actually a better and potentially safer alternative to uranium in the form of thorium.
This got me wondering… Could the long list of nuclear accidents and their consequences have been avoided by using thorium instead of uranium?’.
Thorium sits two places to the left of uranium on the periodic table and possesses comparable properties.
Recommended Video for you:
How Nuclear Reactors Work?
Before we go around throwing accusations at early nuclear scientists for using uranium instead of thorium, let’s take a quick look at how nuclear reactors operate.
Nuclear reactors work by harnessing the energy released during nuclear fission, a chemical reaction in which a larger nucleus splits into two or three smaller nuclei, releasing a large amount of heat and neutrons. The heat energy released during fission is utilized to evaporate water into steam, which is then used to run a turbine and convert thermal energy into electricity or mechanical energy. Simple, right? Well, the entire process is actually much more complicated than it seems and requires a great amount of attention and expertise to manage safely.
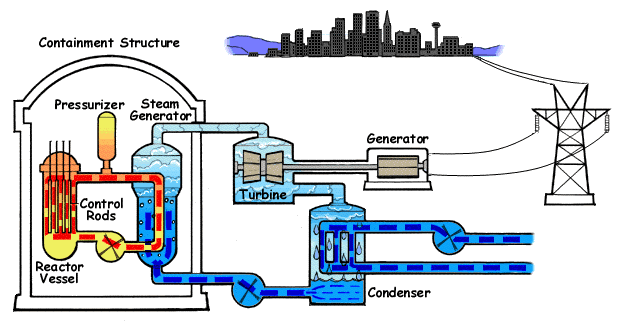
Concentrating on just the chemical side of things, enriched uranium is the substance (nuclear fuel) that undergoes fission in most reactors (some nuclear reactors use MOX, a blend of plutonium and some form of uranium, as nuclear fuel). About 99% of the uranium found on Earth is 238U, the non-fissile isotope, while less than 1% is 235U, the fissile and thus more valuable uranium isotope. Enriched uranium, through the implementation of techniques such as isotope separation, contains an increased percentage of 235U in the overall mixture.
A fission reaction commences when enriched uranium is bombarded with a neutron. Upon impact, 235U starts splitting down into smaller nuclei and releasing high-energy neutrons. The high-energy neutrons are absorbed by other 235U atoms, igniting a chain reaction inside the nuclear reactor. A great amount of thermal energy is released in the process, along with the release of gamma radiation and additional neutrons.
The amount of thermal energy produced depends on the rate of these chained fission reactions. Containing the rate and extent of these chain reactions also draws the line between a nuclear reactor and a nuclear weapon.
The Primary Use Of Nuclear Energy
Long before the development of the first nuclear plant, nuclear energy was originally harnessed for destructive purposes. The Manhattan Project, a weapons research and development initiative commissioned by the US government, pioneered the use of nuclear energy by unveiling the first nuclear weapons. Set up in 1939, the Manhattan Project scared the world with the detonation of the first-ever nuclear weapon (a demonstration known as the Trinity test) in 1945. A month later, the same nation further established the immense destructive potential of nuclear weapons by infamously bombing Hiroshima and Nagasaki, effectively ending Japan’s role in World War II.
The fear of an enemy nation possessing such destructive prowess led other nations involved in World War 2 to start their own individual research into the nuclear domain. What followed was a surge in the production of nuclear weapons, rather than the development of commercial nuclear energy.
The Soviet Union, the UK, France and the PRC, followed by India and Pakistan, have developed nuclear weapons over the years. A few other nations, including North Korea and Israel, are also believed to have developed nuclear weapons. Another country that developed nuclear weapons is South Africa, but they voluntarily destroyed all their nuclear weapons stock and discontinued further production in the 1990s.
Why Uranium?
Enriched uranium might contain an increased percentage of 235U, but it also retains 238U in significant quantities. 238U, upon absorbing one of the high-energy neutrons floating in the reactor, transmutes into 239U before decaying into other radioactive compounds, such as neptunium-239 and plutonium-239. The latter of the two daughter products is what intrigued everyone working on nuclear energy and advancement.
Discovered right around the climax of World War 2, scientists and nations were quick to grasp the destructive potential of plutonium-239. The atomic bomb dropped in Nagasaki was a plutonium-239 bomb called ‘Fat Man’, while the one dropped in Hiroshima was a uranium-235 bomb. Plutonium-239 has the smallest critical mass of all the nuclear fuels, meaning that plutonium can sustain a chain reaction with the least amount of matter.
However, nearly all plutonium is man-made and only a negligible amount can be mined from the Earth’s crust. The only place one finds plutonium-239 in significant quantities is inside a nuclear reactor where uranium-238 is interacting with neutrons. In the US, reactors at the Savannah River site and the Hanford have been established for the production of plutonium-239.
On the other hand, the fission of thorium does not produce plutonium-239 as a byproduct. The thorium fuel cycle (shown above) starts with the transmutation of 232Th into 233U through a series of decays. 233U goes on to play the role of nuclear fuel in these reactors. The thorium fuel cycle also produces plutonium, but the non-weaponizable isotope (plutonium-238). 233U can also be used in nuclear weapons, but the presence of 232Th in the mixture negates its capabilities.
To piece this all together, thorium cannot be weaponized, which meant that research and development of dual-purpose uranium reactors were encouraged (and also funded) in several nations after World War 2. Researchers who voiced their opinions and encouragement for thorium reactors were either ousted from their respective organizations or the ideas were dismissed in favor of uranium’s military uses.
Uranium Vs Thorium As Nuclear Fuel
Apart from being a non-weaponizable nuclear fuel, thorium trumps uranium in several other ways too!
Thorium is three times more plentiful than uranium and does not need to be reprocessed or enriched like uranium-235. Estimations predict that there is enough thorium in the US alone to power the country for another 1,000 years (at the country’s current energy level). Also, thorium ore contains a greater percentage of thorium than the amount of uranium found in its respective ore, making thorium mining more cost-effective and environmentally friendly.
Concerning safety aspects, thorium reactors will generate less nuclear waste than their uranium equivalents. The radioactivity of their nuclear waste is also predicted to diminish to safe levels after a few hundred years, whereas nuclear waste produced by current reactors stays radioactive for thousands of years. This reduces the cost of managing the spent fuel and waste.
More importantly, thorium is a fertile material, but not fissile. Fertile elements do not undergo fission reactions on their own, but upon irradiation in nuclear reactors, they can be converted into fissile material. This implies that thorium reactors can be shut down almost immediately by simply turning off the neutron source.
Final Words
With several known and predicted benefits over traditional uranium reactors, why hasn’t the world seen thorium reactors reign supreme? Because with virtue comes vice!
A lot of research, practical work and testing still needs to be performed before switching to thorium, along with the requirement of capital for licensing and fuel fabrication. Furthermore, the word “nuclear” has become synonymous with uranium, so the possibility of a safer alternative is unheard of by the masses and even by some nuclear domain authorities.
However, there is some hope for change, as many countries have started research and development of thorium-based nuclear reactors. Leading from the front is India, with a three-stage plan to meet 30% of the country’s electrical needs using thorium reactors by 2050, while the world could see its first commercial thorium reactor as early as 2025. Other countries like Canada, China, Norway, the UK, and the USA are also taking a step towards utilizing thorium’s clean and safer nuclear energy.
As for the question asked earlier—could the consequences and damage from those many nuclear disasters have been avoided? Maybe yes. Maybe no… but favoring a proven safer alternative would have definitely helped!
References (click to expand)
- NUCLEAR 101: How Does a Nuclear Reactor Work?. The United States Department of Energy
- Manhattan Project Background Information and Preservation Work | Department of Energy - www.energy.gov
- Nuclear Weapons - Hyperphysics. Georgia State University
- Backgrounder On Plutonium | NRC.gov. The Nuclear Regulatory Commission
- J Ting —. Thorium Energy Viability - Stanford University. Stanford University
- SRS - History Highlights - Savannah River Site. srs.gov
- Thorium-Based Nuclear Reactors - Press Information Bureau. pib.gov.in