Table of Contents (click to expand)
Hawking radiation causes death of black holes by reducing their mass. As black holes lose mass, they emit thermal radiation. Eventually, they lose all their mass and cease to exist.
Eventually, everything dies.
Why should black holes—the magnanimous light-sucking voids of darkness—be an exception?
For starters, black holes are not entirely black, as their name implies. Secondly, black holes do die, and when they do, it happens in a blaze of glory!
By emitting thermal radiation for millions or billions of years, black holes lose their mass, until the point where there is none left. These thermal radiation emissions are what we call Hawking radiation.
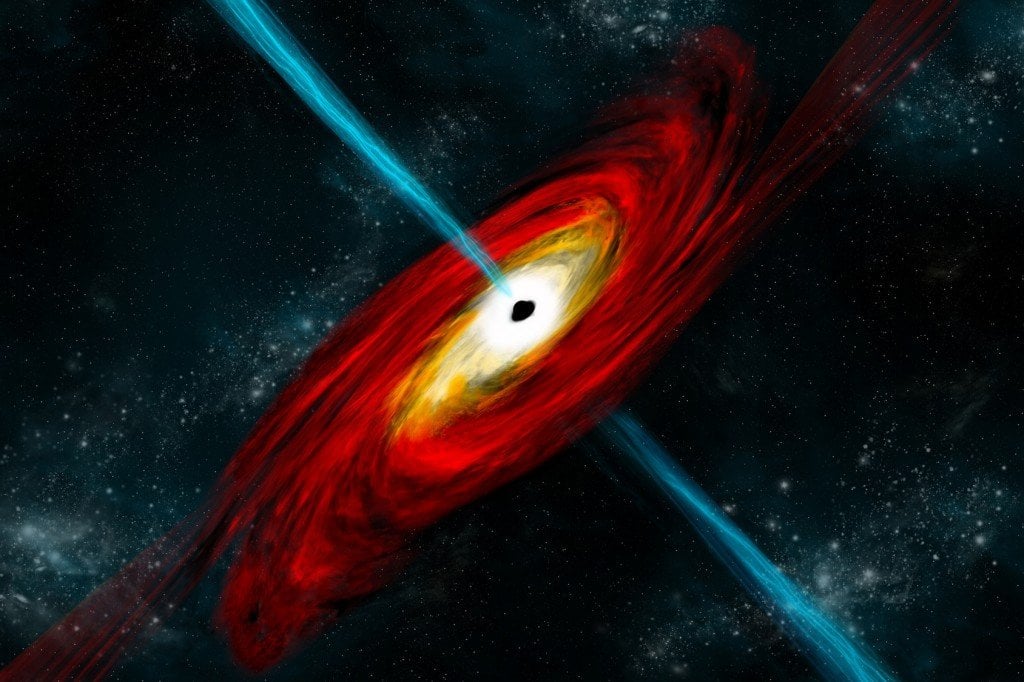
However, wait a second… What?
Isn’t a black hole supposed to suck all nearby matter and electromagnetic radiation? Why has it suddenly begun to emit now? Has it had its fill?
The simple answer is ‘Yes’ if you look at the scenario and apply only classical physics principles. However, on a quantum level, the case is a little different.
Recommended Video for you:
What Is Black Body Radiation?
These are objects that absorb all incident radiation and re-radiate them into the surroundings. These radiated emissions are black body radiations. The intensity of the black body radiation is directly proportional to the temperature of the black body. Now, for a body with a greater mass, the overall temperature of the body is lower, owing to the even distribution of heat energy across the entire body.
Hence, it can be said that only a black body of small mass would have temperatures high enough to emit a considerable amount of radiation.
Now, how do black holes relate to black bodies?
As you might know, black holes are sites of immense gravitational attraction. Classically, the gravitational pull of a black hole is so powerful that nothing, not even electromagnetic radiation, can escape from its grip.
Nevertheless, as you move farther away from the black hole, the gravitational effects grow weaker. Here, quantum physics kicks in. Quantum physics deals with effectively massless particles, so the effects of gravity are not prominent enough to consider. These quantum effects allow the black holes to emit black body radiation.
Quantum Theory
Quantum theory was largely ignored in cases that dealt with gravitational forces, but Stephen Hawking eventually came to an important realization. Quantum fluctuations, at the event horizon(the borderline of no return”, i.e., the boundary at which the gravitational pull of a massive object becomes so great as to make escape impossible.) of a black hole, give rise to virtual particles (exhibit certain properties of normal particles, yet their existence is limited by the uncertainty principle, discussed later in the article ). These virtual particles give rise to the Hawking radiation.
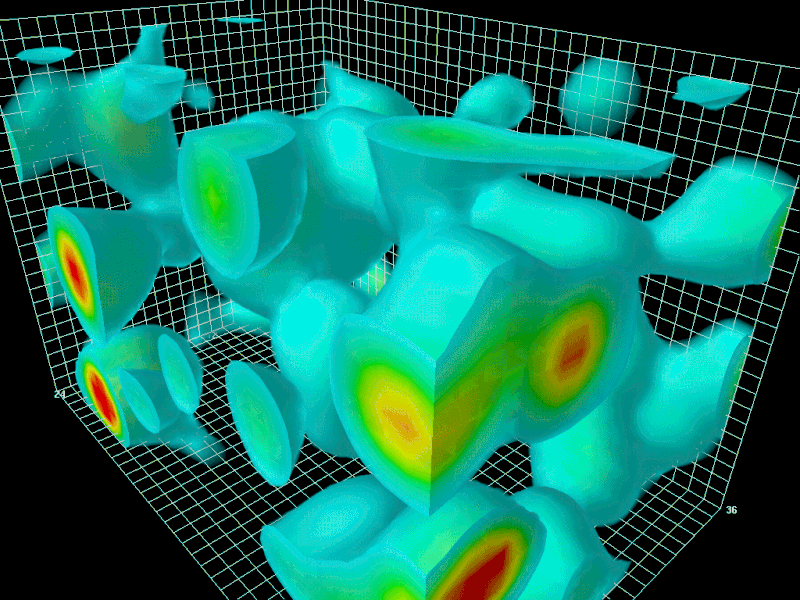
Quantum physics states that empty space is anything but a void.
Now, this might seem hard to digest, but thanks to the uncertainty principle, vacuum space buzzes with particle-antiparticle pairs popping in and out of existence.
Werner Heisenberg, a German theoretical physicist, presented this breakthrough theory of uncertainty. It stated that, for a particle at the microscopic level, having a negligible mass, its position in space and the momentum it possesses cannot be simultaneously measured to high accuracy. This became the basis of modern quantum physics.
However, how does it help our case?
Well, it certainly does. Or should I say, ‘uncertainly’.
Transitioning From Virtual To Real
Heisenberg’s Uncertainty Principle proves the existence of “virtual” particles that cannot be observed directly, according to the law itself, and yet, in every aspect of quantum physics, these particles are fully capable of existing.
To maintain stability in space, these virtual particles occur in pairs of positive and negative-energy particles (particles and antiparticles). These particles are created and annihilated in pairs at exponential rates.
But at what point do they become a reality?
It is that instance, when one of the pair particles, being in close proximity to the event horizon, gets sucked in, while the other escapes. This releases the other particle from the fate of being annihilated. It is at this point, when the escaped virtual particle, becomes a real particle. Owing to the conservation of total energy, the particle that was sucked in, must have negative energy, as an escaped real particle cannot. Negative energy is possessed by antiparticles.
Hence, Hawking radiation is nothing but escaped positive particles. We perceive objects when light bounces off their surface, or when they themselves emit radiation. Similarly, black holes glow slightly with Hawking radiations. That is why a black hole is not entirely black, after all.
Evaporation Of A Blackhole
Hopefully, you’re now convinced that black holes aren’t black after all, but how do they decrease in mass?
Well, if the escaped particle made our black hole “less black”, then the negative particle that got sucked in must have something to do with the reduced mass.
These negative particles have what you would call a negative mass. As unrealistic as this sounds, a negative mass decreases the total kinetic energy of the black hole by emitting photons. As opposed to our classical take on mass, which can be more appropriately stated as “inertial mass’’, negative masses act in a different manner. They repel every other form of mass, unlike a positive mass.
Conclusion
Now that you know how black holes can perish, allow me to add one more thing. The verification of Hawking’s prediction is probably impossible.
This is due to the fact that massive black holes, which do theoretically exist in our universe, have very low temperatures. As mentioned earlier, black body radiations are dependent on the temperature of the body, so observing low-intensity radiations would be impossible. Black holes are themselves, an embodiment of immense amounts of mass, trapped in a small volume; therefore, with strong astrophysical reasoning, it is accurate to say that black holes with small masses should be non-existent!
Astronomers, on April 10 unveiled the first ever photo of these star-devouring monsters scattered throughout the Universe and obscured by impenetrable shields of gravity. The image of a dark core encircled by a flame-orange halo of white-hot plasma and gases, looks nowhere similar to any of the artist renderings, to much dismay of the general masses. Because this time, it’s the real deal. This supermassive black hole, now immortalised by a far-flung network of radio telescopes, is 53.5 million lightyears away in a galaxy known as M87. Having a mass of around 6.5 billion times, that of our Sun, this titan can fit our solar system in its cavity.
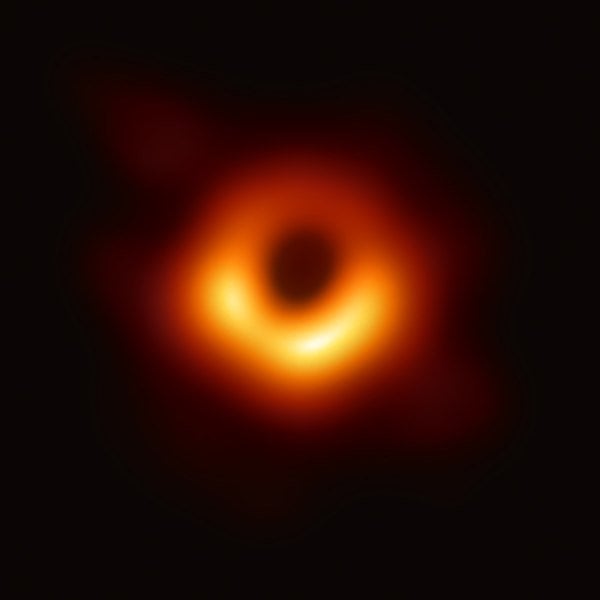
If you feel unhappy with the image captured, you are not alone.
Let me tell you though, to get an image of this resolution, a telescope, the size of our earth is required. That being impractical, scientists used 8 telescopes spread across the globe, to simultaneously observe the radio waves emitted by the black hole. As the earth rotated, the overlapping of captured images helped simulate the outcome, that the earth sized telescope would have rendered. But M87 was not the only black hole they observed. Sagitarrius A star, located around 26 thousand light-years away, at the centre of our galaxy, is currently under observation. Although much closer, this black hole is only 4 million times as massive as our sun and less active at the same time. Hence, we will have to wait a little longer, for our telescopes to observe it completely.
Observing hawking radiations would have been impractical, owing to the humongous size of the black hole and yet, with the current advent, more such discoveries are just around the corner. To learn more, check out this video:
References (click to expand)
- Blackbody Radiation - Hyperphysics. Georgia State University
- Hawking Radiation - UCR Math. The University of California, Riverside
- Spindel, R. (2011). Hawking radiation. Scholarpedia. Scholarpedia.
- Physicists observe weird quantum fluctuations of empty space .... sciencemag.org
- Quantum Fluctuation - Universe-Review. universe-review.ca