Table of Contents (click to expand)
A gravitational slingshot is when a spacecraft uses the gravity of a planet to change its trajectory. The gravity of the planet pulls on the spacecraft, and as the spacecraft swings around the planet, it gains speed. This speed boost can be used to help the spacecraft escape the solar system.
The planets are separated by massive distances. Despite NASA’s most powerful engine at its disposal, calculations showed that Cassini would still be incapable of crossing the ocean of dark space and complete its journey to Saturn. Burning more fuel is not exactly a nifty solution, as rocket fuel is terribly expensive. Behind their glorious qualifications, NASA’s engineers are essentially human beings; they are frugal when it comes to buying a Venti from Starbucks, paying delivery charges or expending rocket fuel. They try to save fuel whenever they can; to achieve this, they often devise idiosyncratic techniques.
For instance, Cassini did reach Saturn, despite the shortage of firepower. If it weren’t for Cassini, we would be oblivious to the gorgeous rings that hug the gas giant. It was one of those strange techniques, mentioned above, that gave Cassini the extra push. The maneuver is called a gravitational slingshot.
Recommended Video for you:
What Is A Slingshot Or Swing-By?
The Sun accounts for roughly 99% of the mass in the entire Solar System. This makes for an exorbitant amount of gravitational pull. This gravitational pull, like terrestrial friction, decelerates probes that travel against it to explore the outer gas giants. However, these probes can be reaccelerated by the same force that bogs them down — the gravity of planets in between can be exploited to “slingshot” these probes and reinvigorate them to finish their set course.
The probes can be flung in the direction of their destination or towards other planets to undergo another or a series of slingshots. The first probe aided by this maneuver was the Soviet’s Luna 3, which was flung by Earth’s gravity towards the dark side of the moon. In fact, when one of the oxygen tanks on Apollo 13 exploded, the captain aborted the landing and decided to slingshot his way around the moon towards Earth. Despite the rapidly diminishing fuel and the insurmountable odds, the slingshot generated enough energy to bring the crew back home safely.
A few decades later, Cassini flew around Venus, Earth and Jupiter before reaching Saturn. Gravity can also be used to decelerate probes that are progressively accelerated by the Sun’s pull as they travel towards the inner, terrestrial planets. It would have been impossible for the MESSENGER to settle in Mercury’s orbit without a couple of swing-bys around Venus, Earth and Mercury itself to lose some of its augmented momentum.
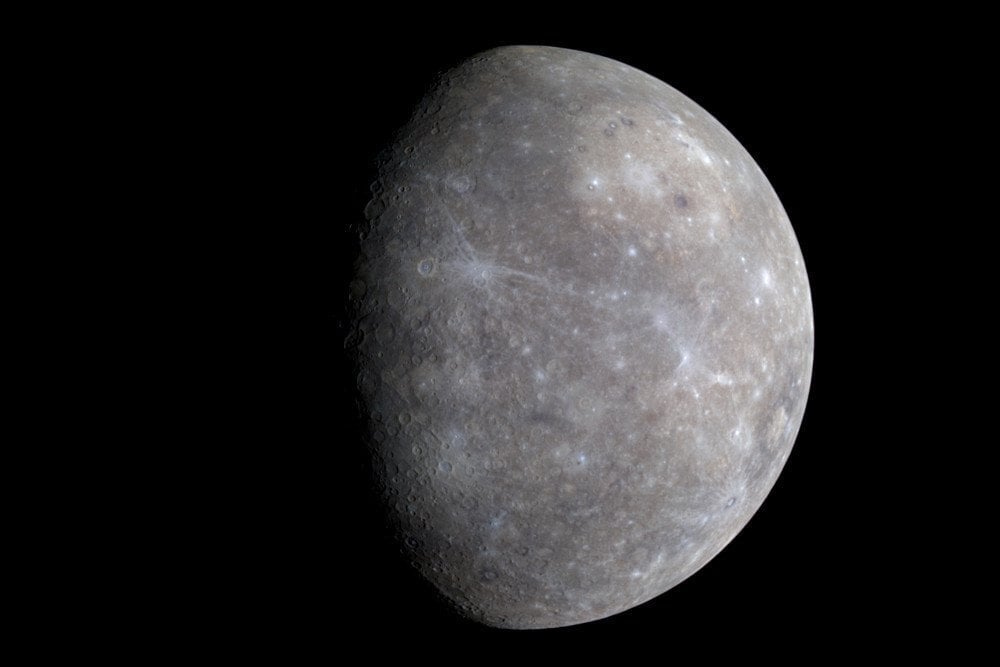
However, there seems to be a slight inconsistency — the maneuver defies the very first commandment of science — the law of conservation of energy. A planet’s gravity digs a steep valley around it, so when a ball enters and rolls down this valley, it gains momentum. That being said, it uses this very same momentum to climb the valley on the other side and escape. The product is sold at the same price it was bought, and the trade produces no profit.
However, the probe does accrue a profit because after exiting the valley, it is accelerated! The system of bodies produces energy out of essentially nothing. The probe accelerates when it travels in the direction of the planet’s motion. The planet cuts in from behind the planet and, as it departs, we observe an abrupt increase in its velocity.
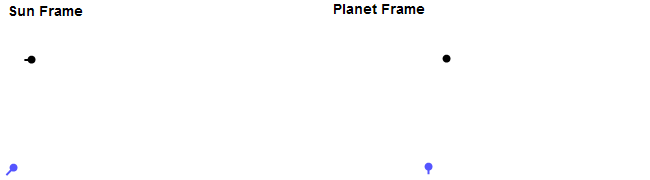
How is this possible?
‘The Probe Steals Some Of The Planet’s Kinetic Energy’
While the planets are stationary from their perspective, they are running in circles from the perspective of the Sun. From the perspective of the planet, or what is formally called its frame of reference, the probe merely changes direction. However, it is from the perspective of the Sun that the probe appears to accelerate or decelerate.
In the Sun’s reference frame, the resulting gain in energy comes at the cost of the planet’s orbital motion. The probe steals some of the planet’s kinetic energy, rendering it immeasurably slowed down. Due to the difference in masses, the retardation is negligible, but even this paltry proportion represents a huge change in the spacecraft’s momentum. In 1979, Voyager 1 slowed down Jupiter’s orbital velocity by roughly 10 to the power of negative 24 kilometers per second. At the same time, the vehicle gained 10 kilometers per second — a dramatic increase in speed!
This finding has a profound implication — if one were to send an enormous army of probes to swing-by a planet, the loss in its orbital energy would be so great that it would eventually collapse into the Sun. Similarly, a probe transfers its energy to a planet when it contacts a planet from the opposite direction. In this case, again, the gain in the planet’s orbital energy is negligible, but the tiny proportion translates to a drastic decrease in the probe’s velocity. This loss allows it to, for example, settle into a planet’s orbit.
Interplanetary Snooker
The procession of events makes it seem like planets and probes are just balls in a game of space snooker. While the planets are the red balls, the probe represents the distinctive cue ball. The cue ball cleverly alters is trajectory by bouncing or deflecting off a few red balls scattered in its path.
However, the efficacy of the shot doesn’t necessarily hinge on the dexterity of the player, which are NASA’s engineers. As the shot can only be played once in a century, its efficacy also comes down to patience – the patience to wait for the right time. The planets do not revolve around the Sun at the same velocity, which renders them misaligned with respect to each other. Now, NASA could either burn excess fuel to cover a larger distance or wait until the planets are conveniently aligned for an economical voyage.
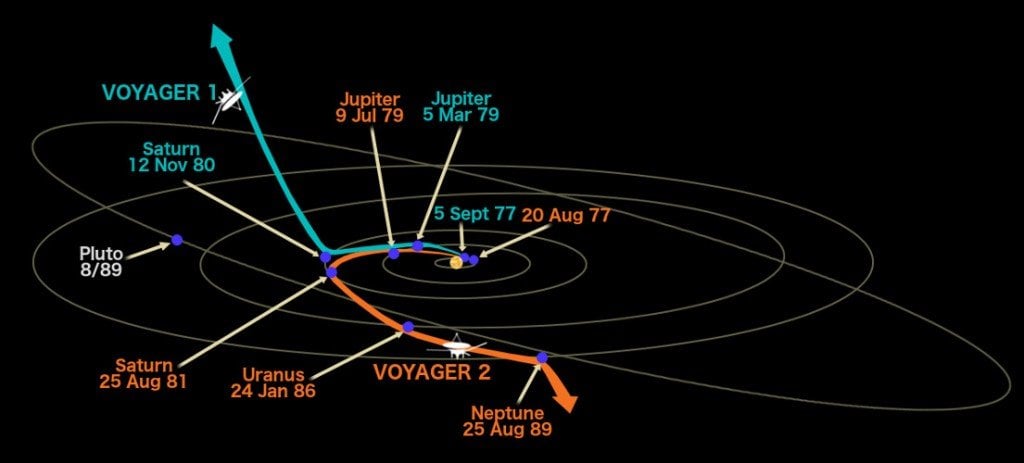
Such an alignment is also liable for gravitational assistance. Voyager 1 and 2 exploited what is called the Grand Tour alignment, an alignment of the outer four planets that occurs only once every 175 years; it will occur next, around 2150. The Voyagers were assisted by every single one of the last four giants to propel further into outer space. However, the right alignment would have been ineffectual if it weren’t for the unprecedented genius of NASA’s engineers, who discerned the angles to the highest degree of precision.
Gravity’s assistance doesn’t just save fuel, but also reduces the duration of a mission. A spacecraft can carry only a limited amount of fuel on its journey; with gravity’s assistance, it achieves extra maneuvering capabilities and course enhancements without expending any extra fuel. Furthermore, the simplicity of the maneuver allows even cheaper, less dynamic rockets to explore celestial bodies or deep space.
Before it became acquainted with Jupiter, Voyager 1 lacked sufficient energy to escape our Solar System. It gained, as mentioned, 10 km/s at Jupiter and an additional 5 km/s at Saturn. If it weren’t for this idiosyncratic technique, Voyager 1 wouldn’t have made it nearly as far – it is now the farthest and the fastest object man has ever propelled into space. It has now receded 18.8 billion km away from us, while Voyager 2, which embarked on a slightly different path, at 15.3 billion km, isn’t far behind.
References (click to expand)
- Gravity assist | The Planetary Society. The Planetary Society
- Gravity assist - Wikipedia. Wikipedia
- Overview | Cassini – NASA Solar System Exploration. The National Aeronautics and Space Administration
- B Casselman —. Slingshots and Space Shots - American Mathematical Society. The American Mathematical Society