Table of Contents (click to expand)
Physicists have been trying to determine the exact nature of dark matter for some time now, but research has implied the existence of a new particle called a dark photon.
In our quest to understand the nature of particles that make up all the matter in our universe, scientists have devised various theories to explain them. People have come up with and discovered numerous particles in the past few decades.
First, it was the atom and its structure, then neutrons, protons and electrons, and now we have quarks, muons, leptons, and more.
Scientists have also studied the forces that interact with such particles. Three of the fundamental forces have their own ‘carrier particles.’ For example, the carrier particle for electromagnetism is the photon. Only gravity is currently left without a carrier particle, though scientists have labelled the corresponding hypothetical particle, the ‘graviton.’
Recommended Video for you:
The Dark Sector
In modern-day physics, The Standard Model of particles vigorously explains the theoretical aspect of smaller-than-atom particles and the fundamental forces (except gravity).
Thus far, it agrees with the results of many particle experiments and accurately predicts most phenomena.
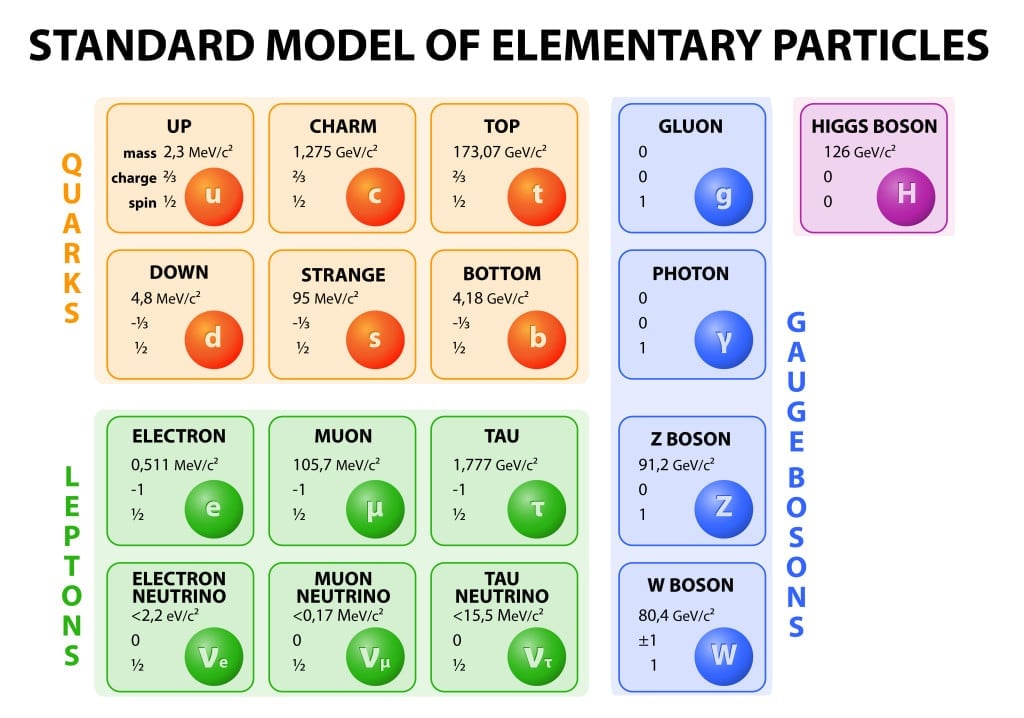
However, the Standard Model is successful in explaining only the fundamental structure of matter. For instance, its mathematics breaks down when gravity is included.
Another aspect where it falls behind is that it does not consider dark matter and dark energy.
Astronomers know that dark matter and dark energy should exist. Without dark matter, all the stars in the galaxy would either fly away or revolve around the galactic center at much slower speeds. Dark energy is needed to explain the current expansion of the universe. Therefore, we are pretty sure they exist (though we don’t know anything about them).
As mentioned, the Standard Model does not include these variables.
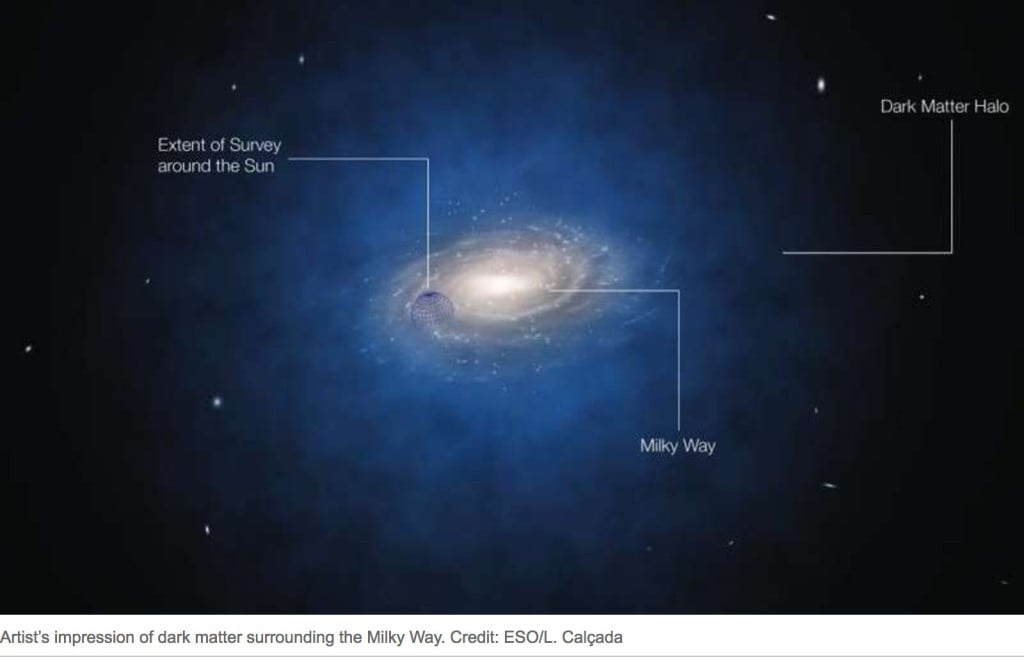
In an attempt to account for dark matter, scientists predict a sort of ‘extension’ to the Standard Model. This extension is called the dark sector. The idea is to account for fundamental particles that could represent dark matter and the other aspects that the Standard Model fails to address.
Also Read: What Is The Standard Model Of Particle Physics?
The Dark Photon
One particular point of interest in this dark sector is a particle called the dark photon. Scientists think that dark matter particles might interact with other dark matter particles via a force similar to electromagnetism.
Scientists therefore established the dark photon as the carrier of this force, just as the ordinary photon is the carrier in the case of electromagnetism.
From theory, dark photons (and other dark matter particles) would only interact with ordinary matter gravitationally. This is because the whole idea of dark matter is to explain the gravitational data obtained from rotating galaxies.
However, physicists think dark photons can switch back and forth with ordinary photons. This phenomenon, although happening at a minuscule level, might be one way in which the dark sector (and by extension, dark matter) could interact with ordinary matter. Termed ‘portals,’ such phenomena could provide the means for us to learn more about dark matter.
Also Read: What Is Dark Matter And How Do We Know It Exists?
Mass Of Dark Photons And Their Production
Since we cannot directly observe dark photons, there is no straightforward way to measure their mass.
Even so, scientists have speculated (in theory) on two cases of dark photon mass: one in which they are massless and the second in which they have some mass. To understand this concept better, suppose that there are invisible cars that cannot be felt or seen (like dark photons). Obviously, there would not be a direct way to measure the mass of these cars.
Now, scientists think that if dark photons have no mass, they can interact with the particles in the Standard Model only indirectly, requiring more than four physical dimensions. This means that to drive our massless invisible cars, we would need to be able to move in more than four dimensions of the universe (three space dimensions and one time dimension). It would be like fitting a square peg into a circular hole or playing 3-D chess, where we can move the pieces vertically and teleport to other parts of the board.
In the case of dark photons with mass, however, they can interact with the Standard Model particles within our four dimensions. Here, we can drive those invisible cars (that have mass) within our current dimensional restrictions. It is like being able to message or call our friends, but not see them physically.
In the case of dark photons with mass, the mathematical formulation does seem to show that it is possible for dark photons to spontaneously turn into ordinary photons and vice-versa, as mentioned previously. Physicists explain this phenomenon by a mechanism called kinetic mixing. The result is that, while possible, this conversion between dark photons and photons can happen only to a small extent when dark photons have a small mass.
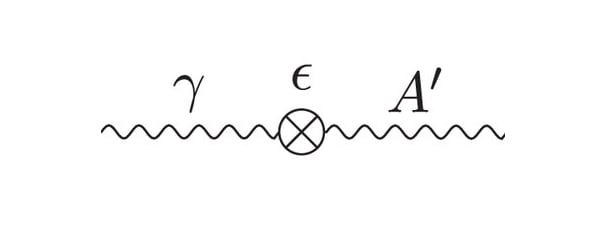
Scientists speculate on the ways in which dark photons are created. Due to their nature of kinetic mixing (however suppressed it may be) with ordinary photons, they may be made where and when ‘virtual photons’ are produced. This usually occurs when two charged particles interact with each other, or when there is particle-antiparticle annihilation.
One of the mechanisms that could create a dark photon is called ‘bremsstrahlung.’ When a moving charged particle (like an electron or proton) comes close to a fixed target (like an atomic nuclei), it could radiate small amounts of dark photons, along with the ordinary photons emitted in bulk.
Another way of producing dark photons is during the annihilation of an electron and a positron, or the joint destruction of quarks and anti-quarks.
Physicists think the production of dark photons can occur during the decay of a class of particles called mesons. For example, pi mesons (or pions) generally decay into gamma rays. However, if mesons (including pions) decay in large quantities, small amounts of dark photons can probably be found.
Detection Of Dark Photons
While directly detecting them would be difficult and unlikely, experimental physicists feel that dark photons would nevertheless leave their mark in high-energy experiments, thus creating an indirect way to observe them. This mark would be in the form of imbalances in the net energy before the start and after the end of the experiment.
Although other Standard Model processes could account for this imbalance, researchers at the ATLAS detector used a machine learning technique (called Boosted Decision Tree) to distinguish the imbalances arising through dark photons from those via other Standard Model processes. While it did not detect disparities coming from dark photons, it did help establish an upper limit for detection that could aid in future experiments.
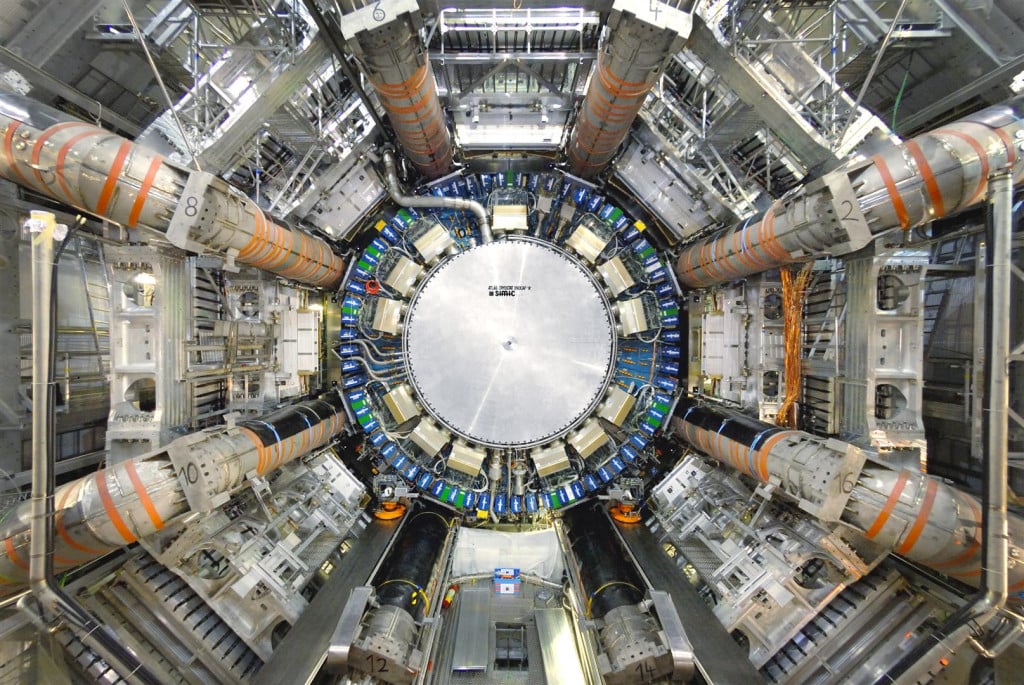
Apart from that, researchers are looking to create dark photons at the Large Hadron Collider via the methods mentioned earlier: meson decay, quark-anti-quark annihilation, etc. One of its significant finds is that it has managed to provide a limit on their mass and the extent of kinetic mixing. Future work would involve detecting dark photons converting into an electron-positron pair, particularly after the upgrade of LHC equipment and more sensitive instrumentation.
Physicists are also trying to detect dark photons via electron-positron annihilation. The BaBar experiment at Stanford Linear Accelerator Center (SLAC) utilizes this process to obtain precise limits on the degree to which it can undergo kinetic mixing and become a Standard Model photon. Future experiments aim to use electron-positron annihilation to study dark photons include the Future Circular Collider.
Other detection methods of dark photons consist of the experiments NA62 and NA64, performed in the Super Proton Synchrotron. The NA62 experiment made use of pion decay. The NA64 experiment looked for evidence of dark photons decaying into the dark sector equivalent of charged particles. In this case, scientists would expect the final energy of the experiment to be well below the initial value, as the decay would take away substantial amounts of it.
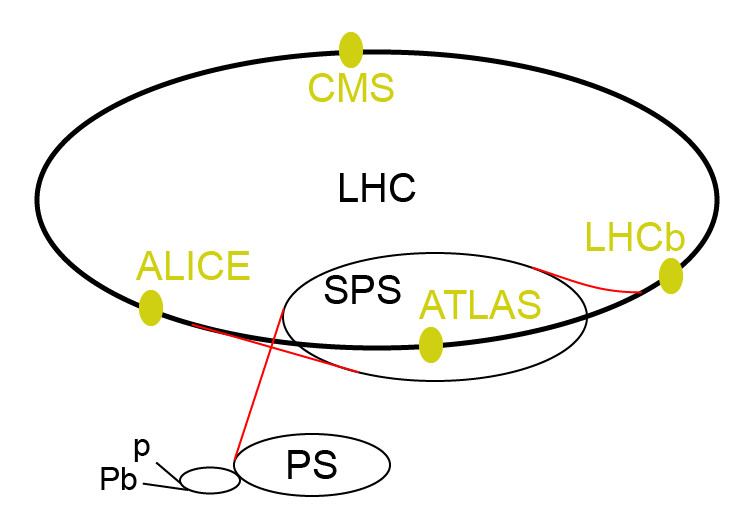
In all of the above experiments, scientists have only managed to obtain limits on the parameters of dark photons, but there has been no direct observation of dark photons. However, these experiments have helped to improve the constraints on those values, particularly the level to which they can undergo kinetic mixing. Future experiments aim to improve this and help us better understand dark photons and dark matter.
Discovering dark photons would be a substantial leap forward in our study of dark matter. Since physicists consider dark photons as one of the potential candidates of dark matter, it will be one of the most significant discoveries in our present age. It will play an essential role in explaining the full nature of our universe, providing a gateway to a whole new world of dark sector particles.
Also Read: Why Is The New Neutrino Map Of The Milky Way Galaxy Significant?
References (click to expand)
- The Standard Model.
- J Wall. Dark Energy, Dark Matter | Science Mission Directorate.
- Dark Sector Physics.
- A Caputo. (2021) Phys. Rev. D 104, 095029 (2021) - Dark photon limits.
- Graham, M., Hearty, C., & Williams, M. (2021, September 21). Searches for Dark Photons at Accelerators. Annual Review of Nuclear and Particle Science. Annual Reviews.
- M Fabbrichesi. (2020) [2005.01515] The Dark Photon.
- Using the Higgs boson to search for dark photons.