Table of Contents (click to expand)
A Geiger counter is a device that can detect radioactive radiation. It works by passing radioactive elements through an inert gas inside the machine, which ionizes the gas. The resulting ions can be easily detected relative to radioactivity itself. This is the working principle of the device.
Developed by the German physicist Hans Geiger, a Geiger counter is an extremely beneficial device that can detect fatal radioactive radiation. Hans Geiger developed the idea around 1912 while working with the meritorious Ernest Rutherford, the same physicist who successfully “split” the atom and discovered the atomic nucleus.
Sixteen years later, he decided to revamp his invention with the help of a fellow student, Walter Mueller. Which is why the device is often known as the Geiger-Mueller counter or tube.
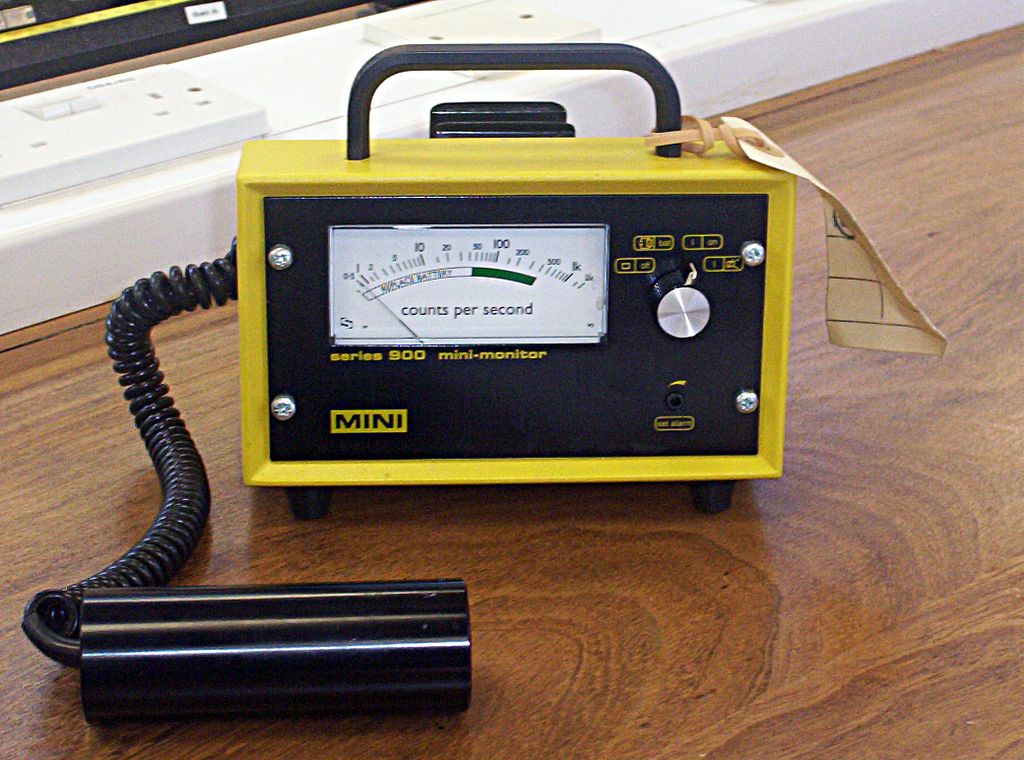
To understand how it works, me must first understand what radioactivity is and why it can’t be measured “conventionally”.
Recommended Video for you:
What Is Radioactivity?
Radioactivity is exhibited by atoms that display a poor hold on the constituents that form their nuclei — protons and neutrons. Thanks to their instability, they become extremely clumsy and occasionally shrug off a few particles here and there. The loss of particles transmutes the nuclei into different chemical elements altogether!
How a group of protons sticks together in such a tight space, despite having such a petulant repulsive force between them, is a mind-boggling feat. This is where the strong force comes into play. The strong force is the strongest fundamental force in the universe, but it only operates at the tiniest of scales. The strong force triumphs over the electromagnetic repulsive force and sticks like-minded protons and neutrons together. The energy required to hold them together is known as binding energy.
However, isotopes — elements that are chemically indistinguishable, but differ in their atomic masses — exhibit some peculiar behavior. A pair of isotopes contains the same number of protons, but a different number of neutrons, or vice versa. A consequence of this is an increase in a nucleus’s size.
Furthermore, an increased size renders the short-range strong force ineffective. At a scale just outside its range, the repulsive force kicks in. At that point, nuclei don’t have enough energy to overcome it and hold their constituents together.
The inconsistency in mass incites instability in an isotope’s nucleus. Atoms naturally covet stability, which in this case comes at the cost of releasing this excess mass. Think about your radioactive nucleus like a crammed seat on a crowded bus. The people sitting on it are protons and neutrons. When an extra neutron tries to snuggle into an already filled seat, it inadvertently displaces a particle on the opposite side, similar to the steel balls of a Newton’s cradle.
Other than mass, nuclei also emit radioactive energy. In a tug-o’-war between the strongest forces in the universe, the strong force gives in and the repulsive force launches a chunk of particles into its surroundings at very high speeds. This process of emission is known as radioactivity and the atoms are said to undergo “decay.”
There are three types of radiation, depending on their behavior in a magnetic or electric field. Alpha particles are the heaviest, containing two protons and two neutrons, thus bending towards a negatively charged plate. Beta particles are 700 times lighter and are mostly composed of electrons, forcing them to bend towards positive electrodes.
Finally, there are gamma rays, which, due to an absence of any kind of charge, travel undeviated in an electric or magnetic field. Just as an electron can move to lower energy states and emit discrete packets of photons, nuclei lose their energy by emitting powerful gamma rays.
The Geiger Counter
The hardship of dealing with radioactive materials is supplemented by the fact that their emissions are invisible and notoriously difficult to detect. This is why we cannot detect them conventionally. One solution is to somehow convert these invisible immeasurable quantities into detectable and measurable ones. This is exactly what a Geiger Counter does.
A Geiger meter passes radioactive elements through an inert gas inside the machine. Due to their polar nature, radioactive particles ionize the gas they are dispersed in. The resulting ions can be easily detected relative to radioactivity itself. This is the working principle of the device.
A Geiger Counter is a metal cylinder sealed in by a ceramic or mica window at one end. The thin films allow the meandering radioactive particles in the surroundings to permeate it easily. Running down the tube is a thin metal wire, usually composed of tungsten. The end of this wire is connected to a large power supply at the other end, which accumulates a large positive charge. This end acts as a positive electrode — an anode. The curved surface of the metal tube acts as the negative electrode — the cathode.
The cylinder is filed with an inert gas, such as Neon or Argon. As radioactive particles pass through, they ionize this gas. Positive and negative ions pop all around the cylindrical tube. Negatively charged electrons are instantly attracted to the anode, while the positive ions are repelled by the large positive charge and flow towards the cathode.
Moreover, as the electrons move down the gas, they collide into more atoms, causing a chain reaction of ionization that produces more ions and electrons. This is called Geiger discharge. Subsequently, many electrons will arrive at the anode, generating a pulse of electricity that is measured on a meter.
Each pulse from the tube is calibrated to a count. Counts per second give an approximation of the strength of the radiation field. Counts can be read by a user via a visual readout. Visual readouts can be either conventional analog meters or electric LCD screens. Different units, such as milli-Roentgens per hour (mR/hr) or micro-Sieverts per hour (uS/hr), are currently used to display the severity of radiation in a given area.
The wire can also be connected to amplifiers and a loudspeaker to generate the famous “clicks” associated with Geiger meters. Cheaper counters can detect both gamma and beta rays, whereas the expensive ones detect alpha rays as well. However, one limitation of using this device is its inability to distinguish between these radiations because the output pulse is of the same magnitude, regardless of the energy of the incident radiation.
Finally, the device’s functioning can be stopped by releasing a gas that attracts the free ions. This process is called quenching and the gas is known as a quenching gas. Halogens are excellent quenchers due to their strong electronegative nature, although a huge resistance can also be recruited to oppose the flow of current.
What Is The Significance Of The Geiger Counter?
Scientists use Geiger Counters to measure radioactive emission because it is extremely dangerous to humans and animals alike. Being in close proximity to a nuclear plant or an accident involving radioactive elements makes one highly prone to its perilous effects.
Radiation rapidly kills organic cells. Even a small dose can cause radiation poisoning, which induces nausea and makes extremely important parts of the body, such as bone marrow and lymph nodes, susceptible to irreversible damage, causing cancer or other autoimmune diseases.
The loss of white blood cells might be treatable, but survival is only probable, not guaranteed. This is why research labs and power plants involving radioactive activity use vital devices such as these to keep track of harmful radioactivity and ensure that all employees are working in a safe, radiation-free environment.